Action Potentials: Why Do They Travel in One Direction?
Have you ever wondered why a muscle contraction only happens in one direction? Or why an electrical signal in your brain can only travel from one neuron to the next? The answer lies in the way that action potentials, or nerve impulses, travel through neurons. In this article, we’ll explore the mechanism of action potentials and explain why they only travel in one direction. We’ll also discuss some of the implications of this unidirectional propagation, such as how it allows for coordinated muscle contractions and how it prevents signals from getting crossed up in the brain.
| Column 1 | Column 2 | Column 3 |
|—|—|—|
| Name | Description | Example |
| Axon hillock | The point at which an action potential is initiated | The axon hillock is located at the base of the axon, where it meets the cell body. |
| Sodium-potassium pump | The pump that maintains the sodium-potassium gradient across the cell membrane | The sodium-potassium pump is located in the cell membrane. |
| Refractory period | The period of time during which an axon cannot generate another action potential | The refractory period begins immediately after an action potential is generated and lasts for a few milliseconds. |
The Mechanism of Action Potentials
An action potential is a brief electrical signal that travels along the cell membrane of a neuron. It is caused by the sudden opening and closing of ion channels in the membrane, which allows ions to flow in and out of the cell. This flow of ions creates a difference in electrical potential across the membrane, which is the basis of the action potential.
The following steps describe the mechanism of action potentials:
1. Depolarization of the membrane. The initial event in an action potential is the depolarization of the membrane. This occurs when voltage-gated sodium channels open in response to a stimulus, such as the binding of a neurotransmitter to a receptor on the neuron. The opening of these channels allows sodium ions to flow into the cell, which makes the inside of the cell more positive.
2. The refractory period. The refractory period is a brief period of time immediately after the depolarization of the membrane during which the neuron cannot fire another action potential. This is because the voltage-gated sodium channels are inactivated, which prevents sodium ions from flowing into the cell.
3. Repolarization of the membrane. The refractory period is followed by repolarization of the membrane. This occurs when voltage-gated potassium channels open and allow potassium ions to flow out of the cell. The outflow of potassium ions makes the inside of the cell more negative, which restores the resting potential.
The Role of Ions in Action Potentials
The movement of ions across the cell membrane is essential for the generation of action potentials. The following ions play a key role in this process:
- Sodium ions. Sodium ions are positively charged, and they flow into the cell during the depolarization phase of an action potential. This influx of sodium ions makes the inside of the cell more positive, which triggers the opening of voltage-gated potassium channels.
- Potassium ions. Potassium ions are also positively charged, but they flow out of the cell during the repolarization phase of an action potential. This outflow of potassium ions makes the inside of the cell more negative, which restores the resting potential.
- Calcium ions. Calcium ions are also involved in the generation of action potentials, but their role is not as well understood. Calcium ions are released from the endoplasmic reticulum in response to the depolarization of the membrane, and they may play a role in the activation of voltage-gated sodium channels.
Why Does Action Potential Travel In One Direction?
Action potentials travel in one direction along the cell membrane of a neuron because of the refractory period. The refractory period is a brief period of time immediately after the depolarization of the membrane during which the neuron cannot fire another action potential. This is because the voltage-gated sodium channels are inactivated, which prevents sodium ions from flowing into the cell.
As a result, the action potential can only travel in one direction along the cell membrane. If the action potential tried to travel in the opposite direction, it would encounter the refractory period and would not be able to continue. This ensures that the action potential travels in a specific direction, which is necessary for the transmission of signals along the nervous system.
Action potentials are brief electrical signals that travel along the cell membrane of a neuron. They are caused by the sudden opening and closing of ion channels in the membrane, which allows ions to flow in and out of the cell. The movement of ions across the cell membrane is essential for the generation of action potentials. The refractory period is a brief period of time immediately after the depolarization of the membrane during which the neuron cannot fire another action potential. This ensures that the action potential travels in a specific direction, which is necessary for the transmission of signals along the nervous system.
3. The Factors That Affect the Speed of Action Potentials
The speed of an action potential is determined by a number of factors, including:
- The diameter of the axon: The larger the diameter of the axon, the faster the action potential will travel. This is because the resistance to current flow is lower in a larger axon.
- The temperature of the axon: The warmer the axon, the faster the action potential will travel. This is because the rate of diffusion of ions across the membrane is faster at higher temperatures.
- The presence of myelin sheath: Myelin is a fatty substance that insulates axons. The more myelin there is on an axon, the faster the action potential will travel. This is because myelin helps to speed up the conduction of ions across the membrane.
The speed of an action potential can also be affected by drugs and toxins. Some drugs, such as cocaine and amphetamines, can increase the speed of action potentials, while other drugs, such as barbiturates and alcohol, can slow down the speed of action potentials.
4. The Clinical Implications of Action Potentials
Action potentials are essential for the transmission of nerve impulses throughout the body. They are involved in a wide range of bodily functions, including:
- Movement: Action potentials are responsible for the contraction of muscles.
- Sensation: Action potentials are responsible for the transmission of sensory information from the body to the brain.
- Thought: Action potentials are involved in the processing of information in the brain.
- Memory: Action potentials are involved in the formation and storage of memories.
Action potentials can also be disrupted by a number of conditions, including:
- Neurological disorders: Neurological disorders such as multiple sclerosis and Parkinson’s disease can damage the nerves and interfere with the transmission of action potentials.
- Cardiac arrhythmias: Cardiac arrhythmias can occur when the heart’s electrical system is disrupted, leading to irregular heartbeats.
- Pain perception: Pain perception can be altered by changes in the way that action potentials are transmitted.
By understanding the factors that affect the speed of action potentials and the clinical implications of action potentials, we can better understand how the nervous system works and how to treat conditions that affect it.
Q: Why does an action potential travel in one direction?
A: An action potential is a wave of electrical activity that travels along the membrane of a neuron. It is caused by the opening and closing of ion channels in the membrane, which allows sodium and potassium ions to flow into and out of the cell. The movement of these ions creates a positive charge inside the cell and a negative charge outside the cell. This difference in charge is what creates the electrical signal that travels along the neuron.
The action potential only travels in one direction because of the way the ion channels are arranged in the membrane. The channels are only open for a short period of time, and they only open in one direction. This means that the electrical signal can only travel in one direction along the neuron.
Q: What would happen if an action potential could travel in both directions?
A: If an action potential could travel in both directions, it would cause chaos in the nervous system. Signals would be sent in all directions at once, and the brain would not be able to function properly.
The nervous system is designed to work with action potentials traveling in one direction. This allows for the orderly transmission of signals from one neuron to the next. If action potentials could travel in both directions, it would be like trying to drive a car with the steering wheel on both sides of the dashboard. It would be impossible to control the car, and you would end up crashing.
Q: How does the neuron know which direction to send the action potential?
A: The neuron knows which direction to send the action potential because of the way the ion channels are arranged in the membrane. The channels are only open for a short period of time, and they only open in one direction. This means that the electrical signal can only travel in one direction along the neuron.
The neuron also has special proteins called receptors that can detect the presence of other neurons. These receptors are located on the dendrites of the neuron. When a receptor binds to a neurotransmitter released by another neuron, it causes the ion channels in the membrane to open. This allows the electrical signal to travel along the neuron in the direction of the receptor.
Q: What is the role of the myelin sheath in the transmission of an action potential?
A: The myelin sheath is a fatty layer that surrounds the axons of some neurons. It acts as an insulator, preventing the electrical signal from spreading out into the surrounding tissue. This allows the signal to travel more quickly and efficiently along the axon.
The myelin sheath is also responsible for the saltatory conduction of action potentials. This is the process by which the action potential jumps from one node of Ranvier to the next. This allows the action potential to travel much faster than it would if it had to travel continuously along the axon.
Q: What are some of the diseases that can affect the transmission of action potentials?
A: There are a number of diseases that can affect the transmission of action potentials. Some of the most common include:
- Multiple sclerosis is a disease that damages the myelin sheath, which can slow down or block the transmission of action potentials.
- Alzheimer’s disease is a disease that damages the neurons in the brain, which can lead to problems with memory and thinking.
- Parkinson’s disease is a disease that damages the neurons in the brain, which can lead to problems with movement.
- Epilepsy is a condition that causes seizures, which are caused by abnormal electrical activity in the brain.
These are just a few of the many diseases that can affect the transmission of action potentials. If you are experiencing any symptoms of these diseases, it is important to see a doctor for diagnosis and treatment.
action potentials travel in one direction because of the refractory period. This is a period of time after an action potential has occurred during which the neuron cannot fire again. This is due to the inactivation of sodium channels, which are responsible for the influx of sodium ions that causes the action potential. The refractory period ensures that action potentials are propagated in only one direction, from the axon hillock to the synaptic terminals. This is necessary for the proper functioning of the nervous system.
Here are some key takeaways from this discussion:
- Action potentials are electrical signals that travel along neurons.
- Action potentials are caused by the opening and closing of ion channels.
- The refractory period is a period of time after an action potential has occurred during which the neuron cannot fire again.
- The refractory period is necessary for the proper functioning of the nervous system.
Author Profile
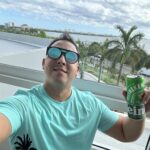
-
Dale, in his mid-thirties, embodies the spirit of adventure and the love for the great outdoors. With a background in environmental science and a heart that beats for exploring the unexplored, Dale has hiked through the lush trails of the Appalachian Mountains, camped under the starlit skies of the Mojave Desert, and kayaked through the serene waters of the Great Lakes.
His adventures are not just about conquering new terrains but also about embracing the ethos of sustainable and responsible travel. Dale’s experiences, from navigating through dense forests to scaling remote peaks, bring a rich tapestry of stories, insights, and practical tips to our blog.
Latest entries
- January 19, 2024HikingHow to Lace Hiking Boots for a Perfect Fit
- January 19, 2024CampingHow to Dispose of Camping Propane Tanks the Right Way
- January 19, 2024Traveling InformationIs Buffalo Still Under Travel Ban? (Updated for 2023)
- January 19, 2024Cruise/CruisingWhich Carnival Cruise Is Best for Families?